Team Members
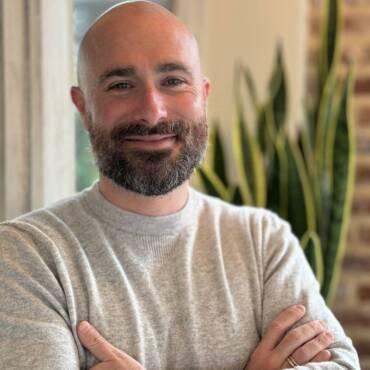
Matteo Alvaro
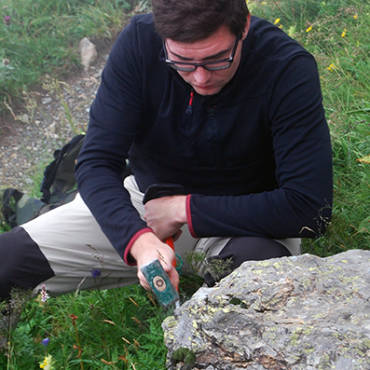
Mattia Gilio
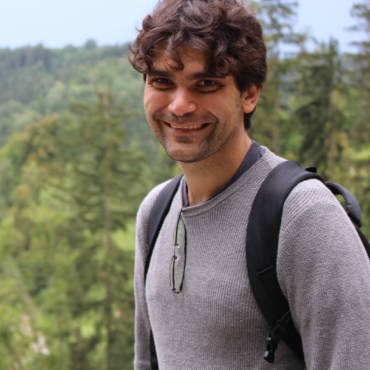
Mattia L. Mazzucchelli
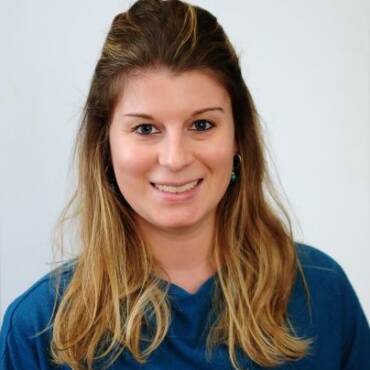
Mara Murri
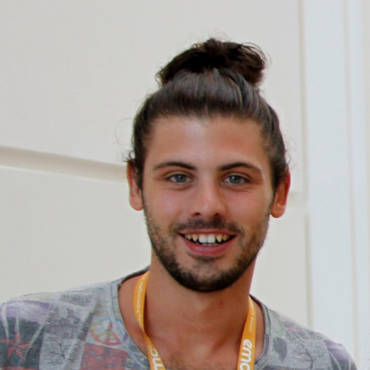
Mattia Bonazzi
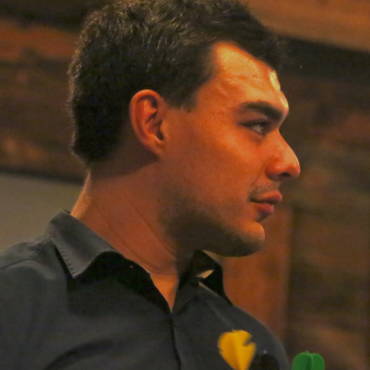
Gabriele Zaffiro
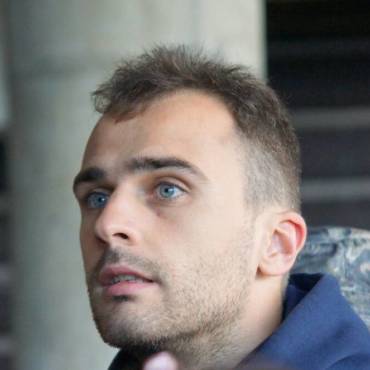
Nicola Campomenosi
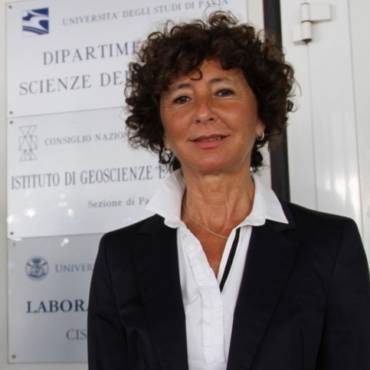
Chiara M. Domeneghetti
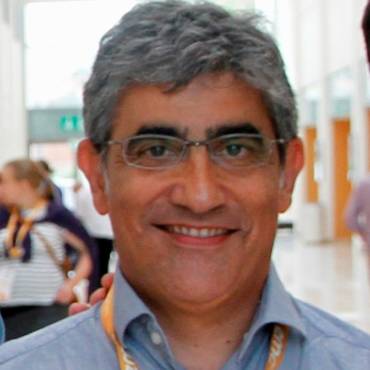
Marco Scambelluri
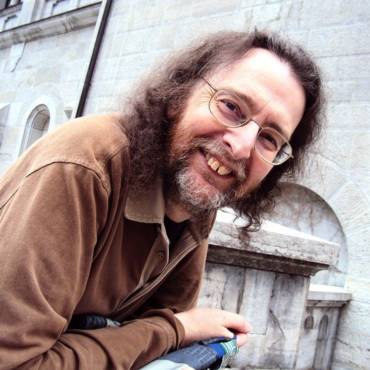
Ross J. Angel
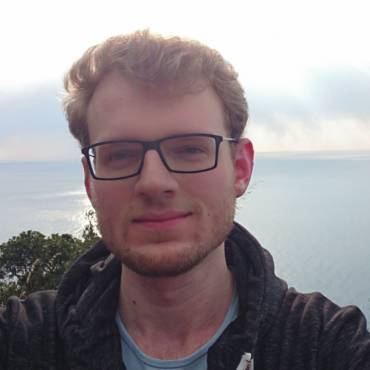
Hugo van Schrojenstein Lantman
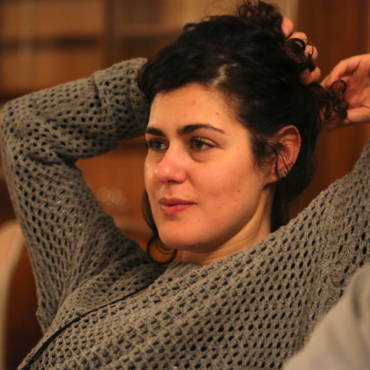
Claudia Stangarone
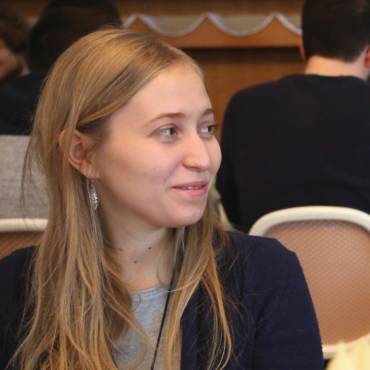
Marta Morana
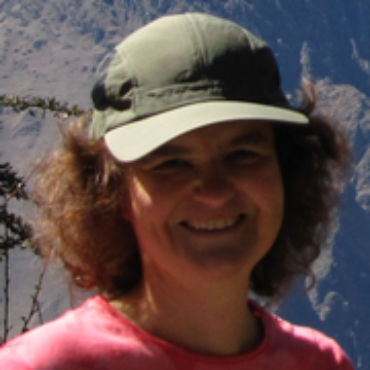
Boriana Mihailova
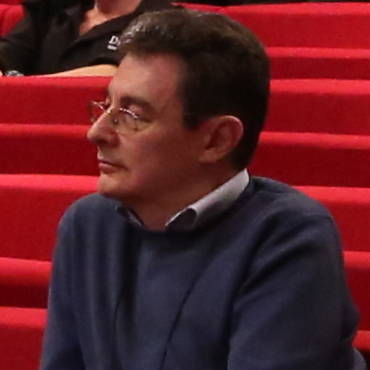
Mauro Prencipe
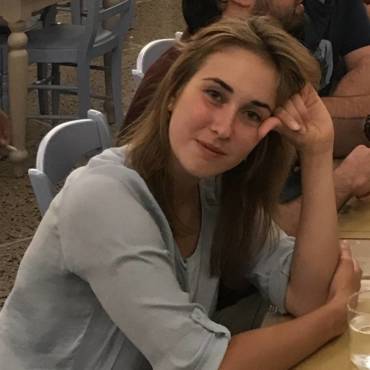
Kira Musyachenko
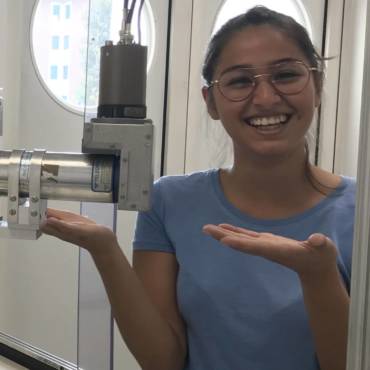
Alice Girani
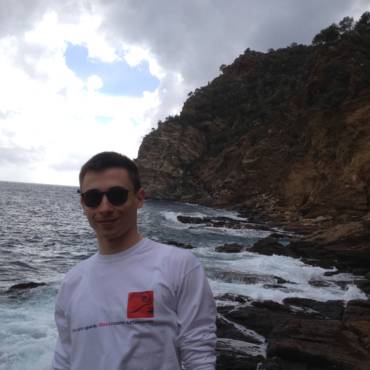
Zeno Geddo
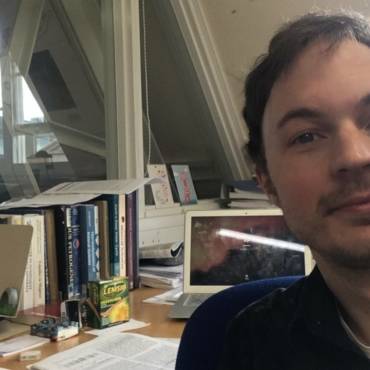
Davide Novella
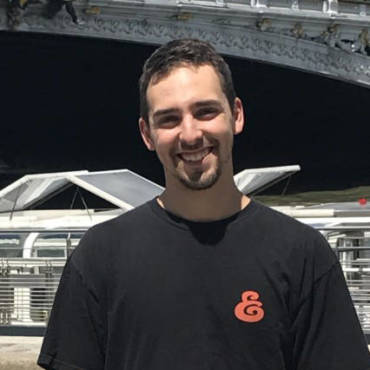
Joseph P. Gonzalez
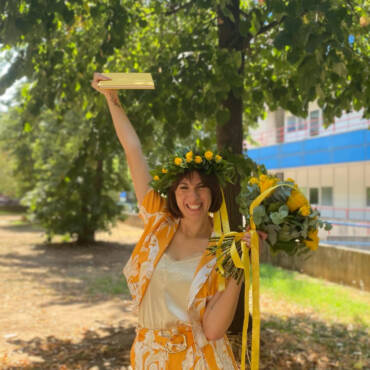
Giulia Mingardi
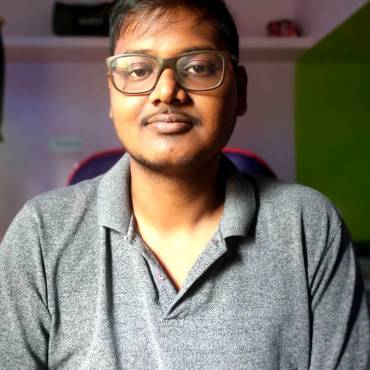
Biswabhanu Puhan
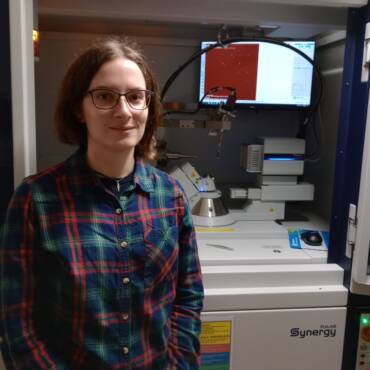
Lisa Baratelli
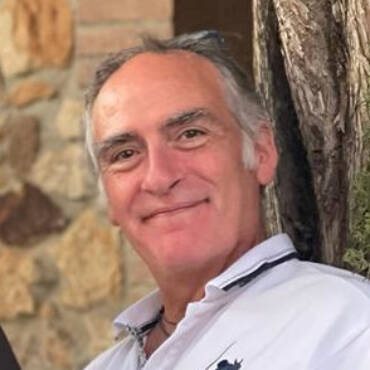
Fernando Cámara
Research project summary
Subduction zones located at convergent plate margins are major geodynamic sites that primarily affect plate tectonics and transport of crustal material deep into the Earth’s mantle. Subduction of one tectonic plate below another plate is accompanied by deformation processes enhancing seismicity and by dehydration/ melting of the subducting plate and overlying mantle, which affect magmatism and volatile (including greenhouse gases) recycling into magmas. Major earthquakes and explosive volcanism directly impact thousands of kilometers of coastal and mountain areas located at present-day and fossil convergent margins. However, the mechanisms attending the downwards transport of crustal material during subduction and its tectonic return back to the surface (exhumation) before/during continent collision, are still poorly understood and controversial. This is because the history of subduction cannot be obtained from real-time geophysical or seismic data which only provide static snapshots of subduction zones today. The study of paleo-geological processes is the key to understanding, modelling, predicting and ultimately ameliorating catastrophic geological events impacting people living near convergent margins. Quantitative understanding the rates and true depths of burial, the thermal regimes of subduction zones and the tectonic processes allied with oceanic subduction and continental collision can only be achieved by determining the pressure-temperature-time-depth (P-T-t-h) histories of Ultra-High-Pressure Metamorphic (UHPM) rocks that have been subducted to pressures greater than 3 GPa and subsequently exhumed to the Earth’s surface.
The fundamental and fascinating challenge is not whether low-density crustal rocks can be subducted to such high pressures. That was established more than 30 years ago, with the discovery of coesite in the Dora Maira massif (Chopin 1984) and the Western Gneiss Region (WGR) of Norway (Cuthbert et al. 2000). The presence of coesite clearly indicates that the rock experienced pressures of 3 GPa or more. The subsequent discovery of other high-pressure minerals including diamond in granulites (e.g. Kotková et al. 2011), clearly indicates such rocks experience pressures in excess of 4 GPa. In the WGR majoritic-garnet crystallization during Caledonian subduction points to subduction pressures of 7-10 GPa (Scambelluri et al. 2010; Spengler et al. 2006). Similar pressures are claimed for Alpe Arami in the Southern Swiss Alps (Green et al. 2010). Traditionally such pressures have been associated directly with depth h, by assuming that the stress state in the Earth is hydrostatic (so P = ρgh, leading to the simple correlation that 30 km depth = 1 GPa of pressure increase). If pressure in the collision zone environment is uniformly hydrostatic, then the pressures inferred from preserved index minerals indicate that the rocks reached depths of 90, 120 or even 300 km. It is then clear that the fundamental and fascinating problem is not that these dense high-pressure rocks exist. The question is how were they exhumed into the much less dense over-lying crustal units in which they are found? A variety of mechanisms involving buoyancy enhancement (e.g. by production of less-dense partial melts, or entrainment with other crustal rocks, or densification of the surrounding subducting material) and mechanically-driven exhumation, have been proposed but all have difficulties in explaining all the field observations. In particular the common absence of major extensional shear zone in the hanging wall of each exhumed unit, the sharp contrasts in recorded pressures over length scales from cm to km, the structural coherency of some UHP terraines (e.g. Zermatt-Saas) and their small volumes, and the absence of structural evidence of the required rapid rate of exhumation, estimated at 3-5cm per year (e.g. Massonne et al. 2007) to match the recorded geochronology and pressure estimates (see Schenker et al. 2015 for a recent review of these issues in the context of the UHP terraines in the western Alps) are all issues of major debate.
The determination of the remnant pressure (or residual pressure) in an inclusion trapped within a host mineral during metamorphism provides an alternative method of barometry by using elasticity theory. It does not rely on the detailed chemistry of the rock, nor on whether chemical equilibrium has been attained at either the local or rock scale. The elastic signal that we can measure in a single inclusion-host pair therefore is not dependent on cation exchange between mineral pairs and is not affected by the exhumation path. Further, mineral inclusions are encapsulated and protected by the host mineral that can be considered as an “inert container”; in many terraines the inclusions are the only preserved evidence of UHPM because of subsequent metamorphic overprinting during the long history of exhumation. Therefore mineral inclusions can be considered as the key witnesses of several geological processes because they carry a signature of processes occurring at great depth and their characterisation can provide a precise picture of the conditions where and when the encapsulation took place.
News and posts
- First high-pressure laboratory experiment on host-inclusion systems
- Elastic Thermobarometry arrives in the Annual Review of Earth and Planetary Sciences
- Can inclusions be symmetry broken?
- What can we learn from trace element and dislocations distribution in Rutile crystals?
- Looking for the next “bible” for diamond research?
- Are you looking for elasticity data for garnet?
- Inclusions in diamonds probe Earth’s chemistry through deep time
- New thermoelastic properties for Zircon
- EMU research Excellence medal to Matteo Alvaro
- Elastic geobarometry: stress, strain, and uncertainty
- Crossing isomekes: elastic geothermobarometry of quartz and zircon in garnet from UHP rocks
- Elastic geobarometry beyond cubic hosts
- EntraPT finally online and published
- about us…
- Under Pressure: High-Pressure Metamorphism in the Alps